Electroplated Tin-Nickel Coatings as a Replacement for Nickel to Eliminate Nickel Dermatitis
This paper is a peer-reviewed and edited version of a paper delivered at NASF SUR/FIN 2013 in Rosemont, Ill., on June 12, 2013.
#research #surfin #nasf
By P. Møller, Technical University of Denmark; J.B. Rasmussen and S. Köhler, Elplatek A/S; and L.P. Nielsen, Danish Technological Institute
Editor’s Note: This paper is a peer-reviewed and edited version of a paper delivered at NASF SUR/FIN 2013 in Rosemont, Ill., on June 12, 2013. A printable PDF version is available by clicking HERE.
Featured Content
ABSTRACT
Nickel dermatitis (skin allergy) is a growing problem in numerous countries. The alarming frequency of sensitization to nickel, especially in the US, has caused nickel to be selected as the “Allergen of the Year” in 2008 by the American Contact Dermatitis Society. Nickel as a coating in contact with skin has already been regulated by the nickel EU directive [94/27/EC] since 1994. In this paper, tin/nickel alloy coatings (66.9 wt% Sn) electrodeposited from a chloride/fluoride-containing alloy electrolyte, are presented as an alternative for both nickel and bright chromium coatings. The main focus will be on the corrosion properties where the following corrosion investigations will be covered: corrosion potential measurements for the different coatings, estimation of corrosion rates for materials in galvanic coupling with tin/nickel coatings, salt spray test, medical tests and immersion test of tin/nickel coatings in artificial sweat.
Keywords: nickel dermatitis, tin-nickel alloy electrodeposits, nickel substitute
Introduction to Nickel dermatitis
Nickel allergy or nickel dermatitis is one of the most common types of contact allergic dermatitis. In many countries, electroplated nickel coatings have been banned in applications involving direct and prolonged skin contact. This is also the case when a nickel substrate or a nickel plated surface is coated with a protective layer of another metal such as chromium, gold, silver or a thin organic coating/paint. The reason is that even small pinholes, scratches or other defects in the protective layer may cause the release of nickel ions by corrosion. This is especially the case when the corrosion is accelerated by a galvanic coupling with other metals and in the presence of a “sweat induced electrolyte,” containing among others chloride, lactate or ammonia ions. Nickel ions form a lactate complex as well as ammonium complexes, which significantly increases the corrosion speed of nickel by reducing the thermodynamic stability of nickel.
The affected skin areas become intensely itchy and may become red and blistered (acute dermatitis) or dry, thickened and pigmented (chronic dermatitis) as a consequence of the allergic reaction.
The European Nickel Directive regulates the use of nickel products that come into direct and prolonged contact with the skin. These limits, known as migration limits, are:
0.2 μg/cm2/week for post-assemblies, which are inserted into pierced ears and other pierced parts of the human body,
0.5 μg/cm2/week for other products intended to come into direct and prolonged contact with the skin.
Medical treatments can reduce the symptoms of nickel allergy. However, once a person has developed a nickel allergy, that person will always have an increased sensitivity towards nickel and the need to avoid future contact with the metal.
Figure 1 shows nickel dermatitis (skin allergy) caused by a bright chromium plated ABS polymer. The thickness of the chromium layer is normally below 1.0 µm and many small defects in the chromium layer make it possible to establish a galvanic coupling with the bright nickel layer below. Sweat which contains ammonia, chloride and organic acids such as lactate forms a chemical complex with nickel and accelerates the anodic dissolution process. The cathode reaction takes place on the chromium layer and can be described as a reduction involving oxygen. The red coloring of the cotton swab indicates that nickel is corroding and is caused by the indicator dimethylglyoxime [Fig. 2 and Ref. (8)].
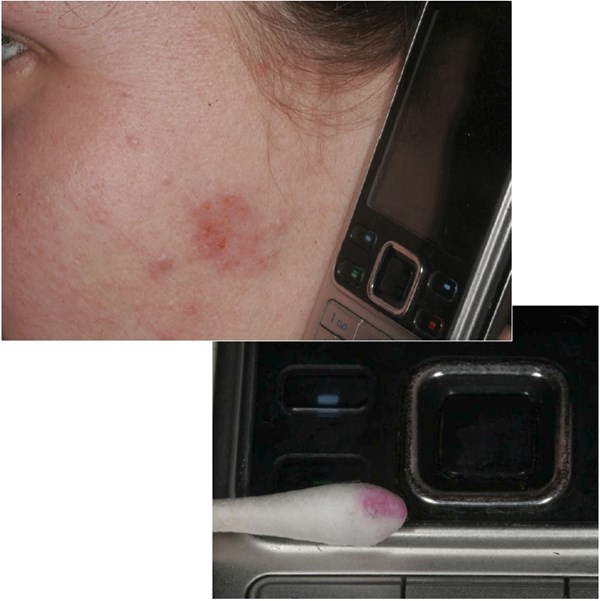
Figure 1 - Nickel dermatitis (skin allergy) caused by a bright chromium plated ABS polymer. The upper photo shows allergic contact dermatitis caused by nickel in a cell phone; the lower photo shows the results of a nickel spot test of the cell phone (Photos courtesy of Dr. Niels K. Veien, Doctor of Dermatology.).
Figure 2 shows a bright nickel-plated paper clip reacting positive (red complex on the swab) on the dimethylglyoxime test (DMG). The chemical reaction between nickel ions and dimethylglyoxime and formation of the red complex is shown. When the color is formed with this test, it can be stated that the nickel release is above 0.5 μg/cm2/week. A nickel release of 0.5 μg/cm2/week corresponds to a corrosion speed of 0.6 nm/week assuming a nickel density of 8.9 g/cm3. Since the Ni-Ni distance is close to 2.5 Å (0.25 nm), a corrosion rate of 0.5 μg/cm2/week corresponds to a corrosion rate of close to two atomic layers of nickel per week. This is an extremely low nickel corrosion rate, which is difficult to measure.8
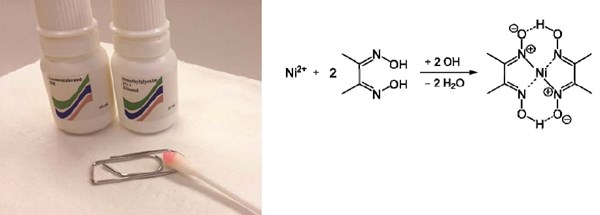
Figure 2 - A bright nickel plated paper clip reacting positive (red complex on the swab) on the dimethylglyoxime test (DMG).
Figure 3 shows the Pourbaix diagram calculated for nickel with a concentration of 10-6 mol/L corresponding to a concentration of 58 μg/L of nickel. For a comparison, nickel concentrations in European drinking water are reported to be generally below 10 μg/L of nickel. Observing the diagram it is not surprising, that nickel is not thermodynamically stable even in water at pH 7.0, especially in the case of nickel where even the dissolution of a few atom layers from a surface can initiate an allergic reaction.
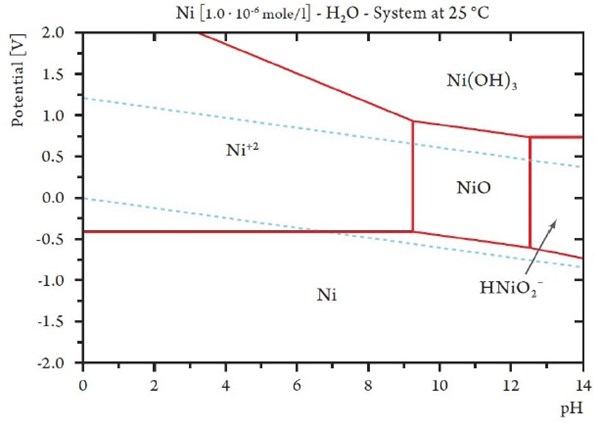
Figure 3 - Pourbaix diagram calculated for nickel with a concentration of 10-6 mol/L corresponding to a concentration of 58 µg/L nickel.
Electroplated nickel coatings are of great technical importance for many industries to prevent corrosion and wear. If nickel is used wisely and not on surfaces intended to be in contact with skin under moist conditions for extended periods, such as eyewear, watches, jewelry, hand tools, etc., nickel will not cause allergic reactions. A nickel allergy usually only develops after repeated or prolonged exposure to nickel-containing surfaces.
Finding replacement coatings for nickel in situations where it may be assumed that there may be prolonged skin contact is clearly a challenge. Electrodeposited copper/tin/zinc alloys are among the few coatings that have been proposed. In the following discussion, it will be demonstrated that tin/nickel coatings are probably a better solution from a materials science point of view, since they have a number of very interesting properties in addition to the already known corrosion resistance. In the following some of these properties will be discussed and reviewed in further detail.
The tin/nickel coating
In 1951, Parkinson published detailed process data describing a process capable of electroplating a deposit of nickel and tin with the composition of approximately 65/35 wt% corresponding to a one-to-one atomic ratio of Ni/Sn, referred to as NiSn alloy. This intermetallic phase of equiatomic nickel and tin cannot be formed by any pyrometallurgical process8 and is not to be found in the phase diagram shown in Fig. 4.
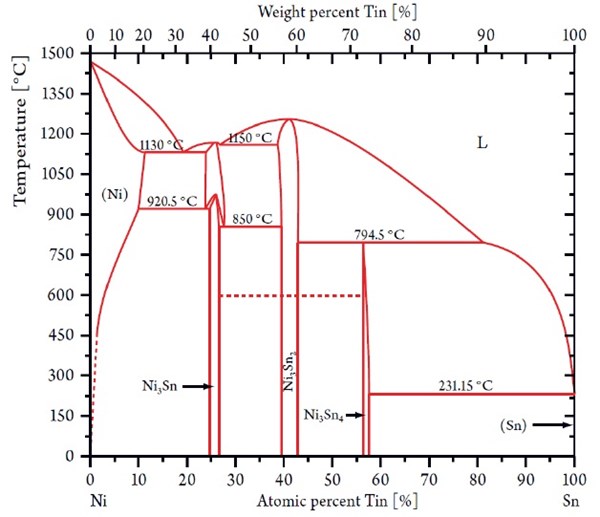
Figure 4 - Nickel-tin phase diagram showing the stable NixSny phases.
Early descriptions explained the existence of a tin/nickel phase as an extension of the composition range of the equilibrium phase Ni3Sn2. It was suggested that this phase extension should have significantly more favored nucleation kinetics at low deposition temperature and the bath resistances suggested by Parkinson, et al.1 Later the structure of an equiatomic tin/nickel deposit was investigated by Lo,2 who also proposed that the electrodeposited tin-nickel alloy could be characterized as Ni3Sn2 grains with the additional tin located at grain boundaries. More recently, Watanabe3 hypothesized that the NiSn formation could be explained by a change of the lattice occupancy probability by nickel atoms.
Most investigations of the crystal structure confirm that the material consists of a hexagonal structure of the type NiAs. This investigation has also been confirmed by other research groups.
It is known that the equiatomic nickel/tin phase will reveal its metastable character when heated above 350°C (662°F), where the nickel/tin phase will decompose into the two stable equilibrium phases Ni3Sn2 and Ni3Sn4 shown in Fig. 4.
Studies published in the British Journal of Dermatology5 reveal that tin-nickel alloys cannot initiate a nickel allergy due to the formation of a very stable oxide passivation layer.2 The nickel release in artificial sweat has been measured to 0.1 μg/cm2/week after long time exposure, a level that is acceptable according to EU regulations, which state a maximum of 0.5 mg/cm2/week. Thus, nickel/tin can be an interesting alternative to nickel coatings in order to minimize risk of nickel dermatitis.
Electrochemical properties of tin/nickel coatings
The effects of chloride, bromide or iodide ions on the passivity of tin/nickel alloys have also been studied. The work showed that the passive state of the nickel/tin alloy was not affected by the presence of halide ions. In another study, also concerning tribocorrosion, it has been shown that the coatings passivate even at a high anodic potential. The Sn/Ni alloy has unique corrosion properties (similar to molybdenum-alloyed stainless steel) and show passivation up to 1200 mVSHE in a 250 mg/Cl-, probably forming a dense surface passivating NiSnO3 oxide. This observation indicates that a galvanic coupling even with gold is probably not able to introduce heavy corrosion on the coating. Given that, gold coatings on top of tin/nickel from a corrosion point of view should be more attractive than gold on top of a conventional nickel coating. Some practical experiments also confirm this hypothesis.
Not even concentrated nitric acid can attack the alloy, which is in agreement with the above mentioned corrosion studies. The visual look is very similar to that of stainless steel and is useful in corrosive environments, where nickel tarnishes or corrodes due to chemical influence such as laboratory equipment of various kinds. It is also useful for the protection of brass or steel parts used in the food sector, but not as food contact materials.
Coatings on brass or tin/nickel cannot replace stainless steel because even small defects in the coating provide risk over time to introduce corrosion of the underlying basis material. Such coatings may be used in applications where normal surfaces like nickel coatings would not be sufficient and where the use of stainless steel is required, for example, gas valves for draft beer equipment (Fig. 5), where contact to carbon dioxide-containing beer contaminants may provide strong corrosion damage to pure nickel coatings.
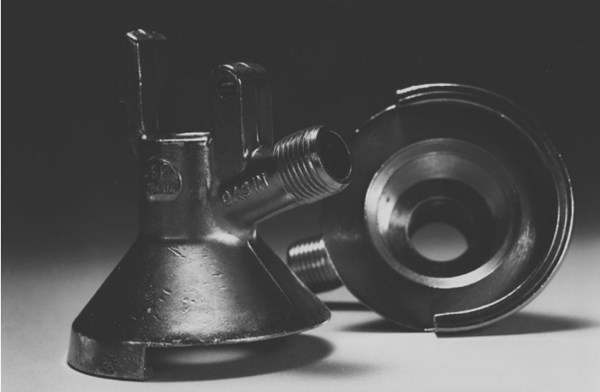
Figure 5 - Gas valve for draft beer produced in hot pressed brass and plated with a tin/nickel alloy (Photo provided by Micro Matic A/S Denmark).
Before examining the tin/nickel coating, the corrosion potential of an as-plated coating was measured in 3% sodium chloride (NaCl) solution corresponding to ocean water. It is remarkable that the corrosion potential for tin/nickel is very close to that of copper and that a bright chromium coating deposited from a Cr+3-based electrolyte shows a lower potential than that of tin/nickel (Fig. 6). It also seems that a galvanic coupling with brass (63/37) is an acceptable solution. The corrosion potential between the mentioned materials seems to be below 50 mV. According to practice, a difference of 50 mV is normally needed to initiate corrosion by a galvanic coupling, which indicates that tin/nickel coatings probably are not very active with respect to corrosion. Furthermore, it is known (to be discussed later) that tin/nickel coatings passivate very easily.
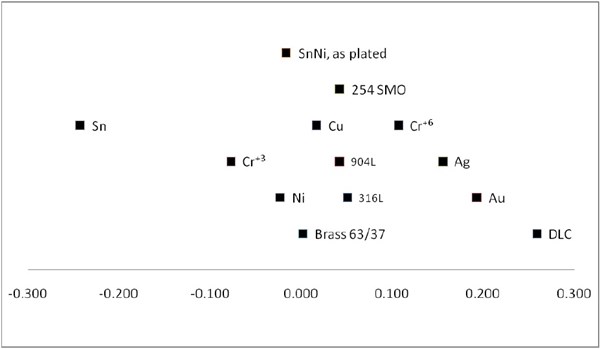
Figure 6 - Corrosion potential or OCP potential measured in VSHE for different materials in a 3% NaCl solution corresponding to seawater. Cr+3 and Cr+6 refer to bright chromium coatings deposited from trivalent electrolytes and hexavalent electrolytes, respectively. DLC is a diamond-like coating containing both diamond and graphite deposited by a conventional PVD process based on a mixture of graphite sputtering and C2H4. Graphite normally shows high potential in such environments and is normally higher than that of gold.
In order to study the passivation properties of the tin/nickel alloy, polarization curves were determined in a test environment of a 3% NaCl buffer based on a sodium acetate solution at pH 6.0 and room temperature (20°C)(Fig. 7). The investigation was carried out with a scan speed of 50 mV/min. The test species were prepared as follows:
- Gold-coated 904 stainless steel sample (4 µm thickness). The basis material was activated by a Wood’s nickel strike.
- Tin/nickel-coated 904 stainless (15 μm thickness) activated by a Wood’s nickel strike. A 0.2 μm (200 nm) gold layer was plated over the tin/nickel coating.
- Tin/nickel-coated 904 stainless (15 μm thickness) activated by a Wood’s nickel strike.
The test sample shown at the top of Fig. 7 was coated with gold at the beginning of the polarization run. During the polarization run, the gold coating was stripped away from the Sn/Ni coating. The picture at top right shows a gold-coated tin/nickel part after exposure to high potential (190 VSHE). It can be seen that the gold coating was stripped away and pits were formed in the 904 SS basis material. The microscopic image at top left shows that the tin/nickel coating was not attacked, although the coating shows cracks around the pit.
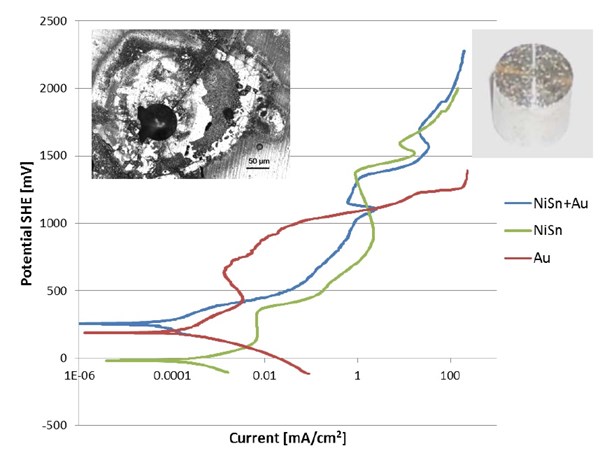
Figure 7 - Polarization curves for Sn/Ni-coated 904 stainless steel (SS) with gold top coating (blue), Sn/Ni-coated 904 SS (green) and a thick gold-coated 904 SS test sample.
The Faraday current measured in connection with gold is caused by the reduction of oxygen and oxidation of the acetate buffer.
O2(g) + 4H+ + 4e- 2H2O (reduction of oxygen in acidic environment).
CH3-COO- + 2H2O 2CO2(g) + 7H+ + 8e- (oxidation of acetate forming CO2).
According to the Pourbaix diagram for gold (Fig. 9) gold cannot be oxidized at a potential below 1000 mV. Around a potential of 1000 mV, the solution almost instantly turned black. The black color is generated by the oxidation of colloidal gold hydroxide Au(OH)3, which can only be formed by anodic oxidation at very high potential (Figs. 7 and 9).
Au + 3H2O Au(OH)3 + 3H+ + 3e-
In the second experiment, a Sn/Ni coating was coated with 200 nm of gold. The corrosion potential (blue line in Fig. 7) was similar to the gold coated 904 stainless steel sample. The anodic part of the polarization curve seems to follow the polarization curve for the pure tin/nickel coating and not the gold test sample (red line in Fig. 7). The Faraday current is probably used for oxidation of acetate and formation of an oxide layer on top of the tin/nickel. It should be stressed that the 200 nm gold layer is filled with defects and offers no protection whatsoever. The formation of the oxide layer on top of the tin/nickel alloy could follow this half-cell reaction or a similar one:
SnNi + 3H2O SnNiO3 + 6H+ + 6e-
A very interesting observation can also be made, at a potential about +1100 mVSHE (Fig. 7). Here, the tin/nickel coating seems to have a corrosion rate below that of than gold, which can only be explained by a passivation or limiting current phenomenon. At this point grey spots start to form and grow on the gold surface (Fig. 8). According to the Au-Pourbaix diagram (Fig. 9), gold is no longer stable, and after a short time, the surface loses its yellow color, and the surface becomes grey (Fig. 8). The gold has been dissolved, but the tin-nickel surface still appears to be smooth.
At an anodic potential around +1350 mV, a rapid increase in current can be observed. After the experiment, the sample was observed under a microscope, and several pits through the layer of tin/nickel into the basis material (904 stainless steel) could be observed. Further examination showed that the surface was covered with small pits (Fig. 7 right insert) and the entire gold layer was removed. The experiment shows that the tin/nickel alloy is has unique corrosion properties superior to the best stainless steel.
The series of photos in Fig. 8 shows how the gold layer is stripped from the tin/nickel coating over time during the anodic polarization of the working electrode. In the third last picture (to the right) the potential is close to +1000 mVSHE and the gold coating starts to corrode, forming colloidal Au(OH)3 which starts to discolor the working electrode. These observations correlate with the blue curve of Fig. 7 for NiSn+Au.
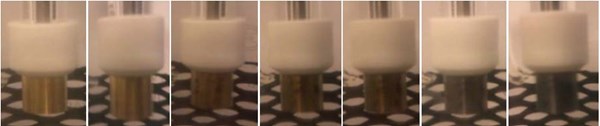
Figure 8 - Photo series showing how the gold layer is stripped from the tin/nickel coating over time during the anodic polarization of the working electrode.
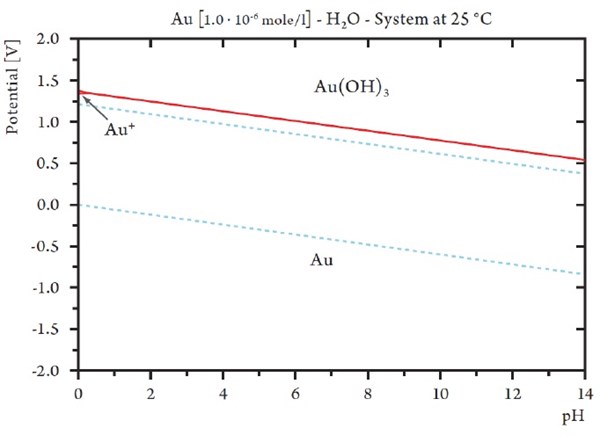
Figure 9 - Pourbaix diagram for gold.
The unexpected discovery was that (except for the pits) the tin/nickel surface was still untouched by corrosion attack. This observation indicates that the tin/nickel coating seems to be more stable than gold during a high anodic potential, but it also seems also more pit resistant than the stainless steel 904 basis material, which has a PREN (Pitting Resistance Equivalent Number) of 36.
Stainless 904 (Table 1) is one of the most resistant stainless steels used commercially. The steel was originally developed to solve corrosion problems in chemical equipment treating sulfuric acid. It also shows high resistance to a wide range of environments. A PREN factor of 35 indicates that the material has good resistance to warm sea water and other high chloride containing environments. High nickel content results in a much better resistance to stress corrosion cracking than the standard austenitic grades. The PREN factor is defined as:
PREN = %Cr + 3.3×%Mo +16×%Ni
Table 1 - Chemical composition of 904L stainless steel.
C | Cr | Cu | Mn | Mo | Ni | P | S | Si |
---|---|---|---|---|---|---|---|---|
0.02 Max | 19.0-23.0 | 1.0-2.0 | 2.0 Max | 4.0-5.0 | 23.0-28.0 | 0.045 Max | 0.035 Max | 1.0 Max |
The copper content adds resistance to sulfuric acid and other reducing acids, particularly in the very aggressive "mid concentration" range. In most environments 904L has a corrosion performance intermediate between the standard austenitic grade 316L and the very highly alloyed 6% molybdenum and similar "super austenitic" grades. The steel is used in processing plants for sulfuric, phosphoric and acetic acids and in seawater cooling equipment.
In Figs. 7 and 8, some indication was shown concerning corrosion potential and passivation. If tin/nickel can passivate, then it can be stated that the pinholes in the gold layer are not critical. This observation opens up the possibility for reducing gold thickness for connectors in the electronic industry or in other application where thick gold layers are applied for avoiding pinholes and later corrosion attack.
As demonstrated in Fig. 10, an experiment coupling gold directly with tin/nickel indicates an extremely low corrosion current. In the figure, the Faraday corrosion current shows that the corrosion speed is very low. A corrosion current about 0.2 μA/cm2 corresponds to a corrosion speed of about 1 micron/year. It should be stressed that part of the corrosion current is used for the formation of the oxide layer on top of the tin/nickel layer (as discussed earlier). Galvanic coupling with DLC layers shows similar results.
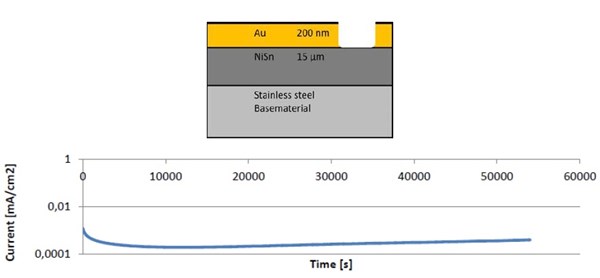
Figure 10 - Corrosion current measured over a period of 55,000 seconds for a galvanic coupling between gold and tin/nickel surfaces.
During the corrosion experiment the galvanic coupling potential was recorded (Fig. 11). From this experiment it can be observed that the coupling potential during the corrosion increases, which clearly indicates that the anodic material passivates. This observation is in good agreement with practice, where the combination of tin/nickel and gold already is in use with good results.
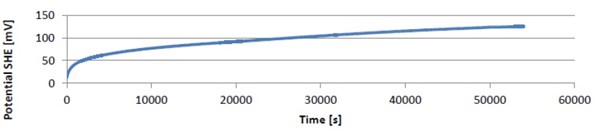
Figure 11 - The development of the coupling potential by coupling of gold and tin/nickel with the same area during 55,000 sec. The curve shows a slow increase in potential, apparently stabilizing around 150 mV. This indicates the formation of an oxide layer on top of the tin/nickel coating.
Conclusion
In conclusion it has been shown that the tin/nickel alloy has extremely interesting anti-corrosion properties enabling a much thinner gold layer when compared to the traditionally applied Au/Ni contact system.
References
1. N. Parkinson, J. Electrodep. Tech. Soc., 27, 129 (1951).
2. C.C. Lo, J. Appl. Phys., 51 (4), 2007 (1980).
3. T. Watanabe, Nano-plating, 17, Elsevier, 2004; pp. 630–640.
4. M.S. Jellesen & P. Møller, Plating and Surface Finishing, 92 (10), 36 (2005).
5. C. Lidén, T. Menné & D. Burrows, British Journal of Dermatology, 134 (2), 193 (1996).
6. IPCS (1991) Nickel. Geneva, World Health Organization, International Programme on Chemical Safety (Environmental Health Criteria 108)(1991).
7. Elplatek A/S, Patent: Corrosion resistant and nickel allergy-free coating combination produced by electroplating for replacing a bright chromium coating based on hexavalent chromium and nickel coatings; http://www.elplatek.dk/ .
8. P. Møller and L.P. Nielsen, Advanced Surface Technology - A Holistic View on the Extensive and Intertwined World of Applied Surface Engineering, ISBN 978-87-92765-17-18; http://astbook.com/.
RELATED CONTENT
-
Cyanide-Free Electroplating of Cu-Sn Alloys
This paper is a peer-reviewed and edited version of a presentation delivered at NASF SUR/FIN 2012 in Las Vegas, Nev., on June 13, 2012.
-
Federal Courts Stay Implementation of OSHA COVID-19 Rules
In response to a backlash of litigation, multiple federal courts have blocked implementation of OSHA and other new vaccine mandates for general industry, the healthcare industry and federal contractors.
-
Mechanical Properties of Electroformed Metals
In 1996, the AESF held its highly regarded electroforming course, prepared by Ron Parkinson for presentation by The Nickel Development Institute (NiDI) and the AESF. What follows is a slightly modified excerpt, specifically on the mechanical properties of electroformed metals. Much of this information has withstood the test of time, and gives a perspective of this technology at the turn-of-the-century.