NASF/AESF Foundation Research Project #121: Development of a Sustainability Metrics System and a Technical Solution Method for Sustainable Metal Finishing - 10th Quarterly Report
This NASF-AESF Foundation research project report covers the tenth quarter of project work (July-September 2022) at Wayne State University in Detroit. This report period covered the development of a significantly enhanced sustainability performance improvement methodology, which (i) allows the evaluation of any number of technology candidates, and (ii) identifies optimal solution(s) through assessing the combined benefits obtained by adopting multiple technologies under various design, manufacturing, cost and technical constraints. The methodology was successfully tested using the data from the five plating facilities.
#nasf #sustainability
by
Yinlun Huang*
Department of Chemical Engineering and Materials Science
Wayne State University
Detroit, Michigan, USA
Editor’s Note: This NASF-AESF Foundation research project report covers the tenth quarter of project work (July-September 2022) at Wayne State University in Detroit. A printable PDF version of this report is available by clicking HERE.
Featured Content
It is widely recognized in many industries that sustainability is a key driver of innovation. Numerous companies, especially large ones who made sustainability as a goal, are achieving clearly more competitive advantages. The metal finishing industry, however, is clearly behind others in response to the challenging needs for sustainable development.
This research project aims to:
- Create a metal-finishing-specific sustainability metrics system, which will contain sets of indicators for measuring economic, environmental and social sustainability,
- Develop a general and effective method for systematic sustainability assessment of any metal finishing facility that could have multiple production lines, and for estimating the capacities of technologies for sustainability performance improvement,
- Develop a sustainability-oriented strategy analysis method that can be used to analyze sustainability assessment results, identify and rank weaknesses in the economic, environmental, and social categories, and then evaluate technical options for performance improvement and profitability assurance in plants, and
- Introduce the sustainability metrics system and methods for sustainability assessment and strategic analysis to the industry.
This will help metal finishing facilities to conduct a self-managed sustainability assessment as well as identify technical solutions for sustainability performance improvement.
1. Student participation
Abdurrafay Siddiqui a Ph.D. student in the PI’s group, conducted research on this project in this reporting period. The student was financially supported mainly by the PI’s NSF grant, and partially by this AESF research project in the summer.
2. Project activities and progress
In the last quarterly report covering the project period from April 1 to June 20, 2022, we reported a case study on the sustainability evaluation of five electroplating facilities. Based on the finding of sustainability performance deficiencies, we identified two technologies for performance improvement: Tech 1 (T1) - The Chemical Concentration Optimization (CCO) Technology and Tech 2 (T2) - The Optimal Water Use and Reuse (OWUR) Technology. Each technology was independently evaluated quantitatively for its capacity for facility performance improvement.
In this reporting period, we developed a significantly enhanced sustainability performance improvement methodology, which allows us to (i) evaluate any number of technology candidates, and (ii) identify optimal solution(s) through assessing the combined benefits obtained by adopting multiple technologies under various design, manufacturing, cost and technical constraints. The methodology was successfully tested using the data from the five plating facilities.
2.1 Optimal technical solution method for sustainability
The solution method contains nine major steps that are described below:
Step 1. Define a system boundary and investigation scope. In this case, the plant’s sustainability performance needs to be evaluated, and if weaknesses are identified, a solution needs to be identified.
Step 2. Determine the plant’s sustainability status and set an improvement goal.
- Select a set of economic, environmental, and social indicators from the sustainability
- metrics system, i.e., Ei’s, Vj’s, and Lk’s, where i ∊ IE, j ∊ IV, k ∊ IK.
- Conduct sustainability assessment of the plant.
- Analyze the assessment result and identify the weaknesses that need to be overcome.
- Provide a budget plan and spending limit data by the plant management.
- Set a performance improvement goal in different sustainability categories.
Step 3. Develop technology sets based on the given technology candidates.
- Based on the identified weaknesses, the plant needs to provide a number of technologies as candidates for possible adoption. Let’s assume that there are N candidate technologies (namely T1, T2, …, TN), and each technology’s adoption cost should also be collected.
- For the N candidates, generate 2N - 1 technology sets, which contain different technology combinations. A general formula for calculating the number of technology candidates in each set is
, where k is the number of candidates in a set.
- Determine the cost for the use of each technology set (including the license fee, technological support fee, etc.). Negotiation on fees may be needed, as a technology vendor may agree to offer discount if more technologies are adopted by the plant.
Step 4. Determine affordable technology sets. Based on the company’s budget limit, eliminate those
unaffordable ones from a total of 2N - 1 sets. Let’s assume that there are M sets left (M ≤ 2N - 1). If M = 0, then go to Step 7; otherwise, continue.
Step 5. Conduct sustainability assessment of M affordable technology sets.
- Use the same sustainability indicators as those used in Step 1 to evaluate the sustainability performance improvement capacity of each technology set.
- Calculate the technology set’s ability to improve the plant’s economic, environmental and social sustainability performance.
Step 6. Identify optimal technology sets for the plant to achieve its sustainability goal. This can be accomplished by following the sub-steps below.
- Check each of M technology sets’ capability of achieving the economic sustainability goal and delete all those incapable ones. If no technology set is left, then go to Step 8; otherwise, continue.
- If there are some technology sets left, then check each of the remaining technology sets’ capability of achieving the environmental sustainability goal and delete the incapable ones. If no technology set is left, then go to Step 8; otherwise, continue.
- If there are some technology sets left, then check each of the remaining technology sets’ capability of achieving the social sustainability goal and delete all those incapable ones. If no technology set is left, then go to Step 8; otherwise, continue.
- Calculate the overall sustainability value of each of the remaining technology sets and then go to Step 9.
Step 7. Go to Steps 2 - 4 to adjust the budget commitment and/or identify other alternative technologies
by the plant management; otherwise, go to Step 9.
Step 8. Go to Steps 2 - 5 to adjust the preset sustainability goal by the plant management; otherwise, continue.
Step 9. Generate a report. If it is from Step 7, then no solution is identified for sustainability performance improvement under the given conditions and constraints. Otherwise, there will be at least one technology set identified, which is optimal. In the case of more than one solution, all of them are optimal. The plant management can select one most suitable for adoption, as those technology sets may have different cost requirements, and the detailed sustainability performance may have some specific differences.
2.2 Case study
The sustainability evaluation of these facilities can be seen in past project reports (Section 5). Three technologies were identified for five electroplating facilities; they are: Tech 1 (named T1) - an in-process chemical recovery technology that adds a static rinse tank immediately after the electrocleaning tank and then recycles lost chemical solution back to the presoak tank; Tech 2 (T2) - a comprehensive water reuse technology that monitors the cleanness of used water and reroutes water flow among different rinse tanks within the process; and Tech 3 (T3) - a hoist scheduling technology that focuses on waste reduction while optimizing the production rate. The data for these three technologies was collected from a number of previous studies in the PI’s lab.1-4 The sustainability goals set by each facility are listed in Table 1.
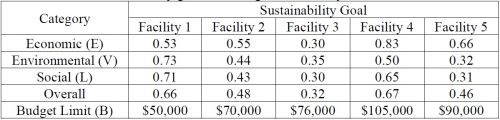
Table 1 - Sustainability goals and budget limits set by individual facilities.
The three candidate technologies can form seven technology sets as follows: (i) three sets, one technology each (i.e., {T1}, {T2}, {T3}), (ii) three sets, two technologies each (i.e., {T1, T2}, {T1, T3}, {T2, T3}) and (iii) one set containing all three technologies {T1, T2, T3}. Every technology set was evaluated using the same sustainability indicators as those for process performance assessment. Their sustainability performance improvement capacities are listed in Table 2.
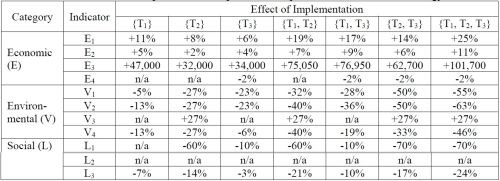
Table 2 - Indicator-based performance improvement capacities of individual technology sets.
2.2.1. Facility 1. The facility’s budget limit ($50,000) is used first to check the technology sets’ adoption feasibility. As shown in Table 3, Sets 4 to 7 are immediately removed because they were all not affordable. For the remaining three sets, their economic sustainability performances after using the technology sets are evaluated. (See the data in the 4th column under E(T:P)). As the preset goal for economic sustainability (E) is 0.53 (See Table 1), Sets 2 and 3 should be removed as they are incapable of making the plant achieve its economic sustainability goal. To this point, Set 1 is the only one left for further assessment. However, this technology can improve the environmental sustainability (V(T:P)) to only 0.68, which is below the plant’s expectation (See the plant’s goal of 0.73 in Table 1). Therefore, this technology set cannot be selected either. Consequently, no technology can be selected under the given budget to satisfy the sustainability goal.
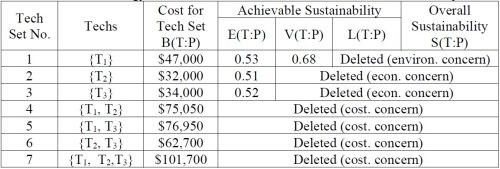
Table 3 - Technology evaluation and solution identification result for Facility 1.
2.2.2. Facility 2. Its budget limit is set to $70,000. Using the solution method, we can find that Sets 1 and 6 are the solutions for the facility to achieve its sustainability goals. The specific economic, environmental, social and overall sustainability performance date after using each of the two technology sets are listed in Table 3. As shown, Set 6 (using both T2 and T3) offers a better sustainability performance, but it costs more. The facility management can balance their interests in technology selection.
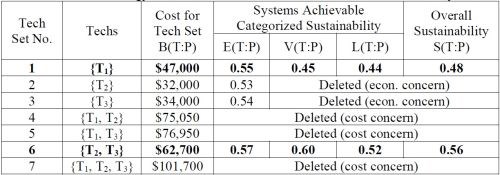
Table 4 - Technology evaluation and solution identification result for Facility 2.
2.2.3. Facilities 3 to 5. The budget limits set by Facilities 3, 4 and 5 are $76,000, $105,000 and $90,000, respectively. Using the same solution method, the facilities identified different solutions. Tables 5 to 7 detail the identified solutions associated with the achievable sustainability performance data.
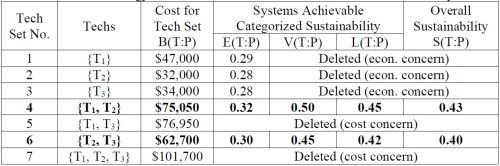
Table 5 - Technology evaluation and solution identification result for Facility 3.
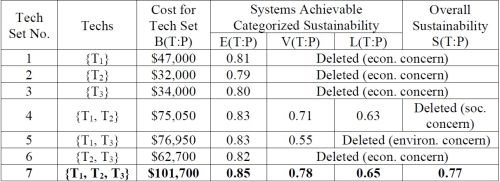
Table 6 - Technology evaluation and solution identification result for Facility 4.
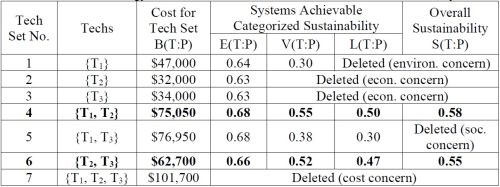
Table 7 - Technology evaluation and solution identification result for Facility 5.
2.2.4. Summary. The solution identification and sustainability performance for each of the five facilities shown in Tables 3 to 7 are summarized in Table 8. As shown, no technical solution is identified for Facility 1, but each of the other facilities can adopt the identified solution(s).
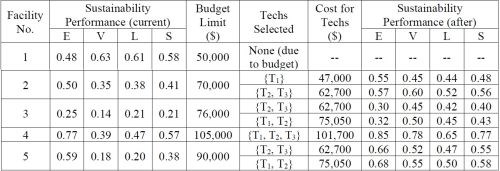
Table 8. Summary of technology solution identification result for five facilities.
2.2.5. Further discussion on budget setting. Table 8 shows that there is no technical solution suitable for Facility 1. Table 3 provides detailed information. Since the budget limit was $50,000, Sets 4 to 7 were immediately excluded. The remaining three sets (Sets 1 to 3) were all unable to improve the facility’s sustainability performance to the level that the facility expected. It is better for the facility to consider an increment of its budget commitment, if the preset sustainability goals are to be maintained. Here, we assume that the facility increases its budget to $100,000. With this new budget limit, one solution is identified, which is Set 4 (i.e., the use of both technologies T1 and T2. The total cost for this technology adoption would be $75,050, which is well within the budget limit. Table 9 provides the details.
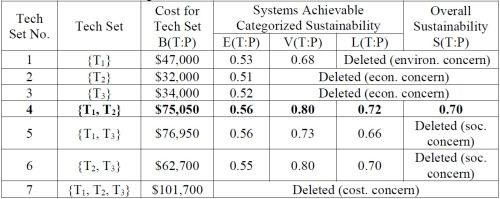
Table 9. Technology evaluation and solution identification result for Facility 1, if the budget limit is increased from $50,000 to $100,000.
2.3. Publication and presentation activities
The PI published an article, titled “Toward Dynamic Sustainability Assessment in the Digital Age”, in a Springer journal, Clean Technologies and Environmental Policy,”5 The development of this article was in fact motivated by this AESF project and the attendance at the NASF SUR/FIN conference in Detroit, Michigan in November 2021. From the conference, it is evident that Industry 4.0 has started to make a very positive impact in the small and medium sized manufacturers (SMMs). In the digital age, the data-rich environment, particularly with ample real-time data accessible, offers the best opportunity for developing a knowledge base that contains key information revealing intrinsic relationships between a plant’s dynamic behavior and sustainability performance change. The PI believes that dynamic sustainability assessment will help the metal finishing industry advance its manufacturing activities for a more sustainable future.
As the recipient of the AIChE Lawrence K. Cecil Award, the PI will deliver a plenary speech, titled “Life-Cycle-Based Multiscale Sustainability: Challenges and Opportunities in the Era of Industry 4.0,” at the AIChE Annual National Meeting in Phoenix, Arizona, November 13-18, 2022. In the speech, the research progress of this AESF research project will be reported as well. At the same conference, the PI’s student, Abdurrafay Siddiqui, will present two papers, titled “Plant-wide Digital Twinning of Surface Finishing for Sustainable Manufacturing,” and “Technology Assessment and Impact Analysis for Life Cycle Based Sustainability Performance Improvement of Process Systems.”
3. Plan for the 11th quarter of the project
The case study discussed here demonstrates the methodological efficacy. The solution method is general and systematic. It can be used for any plant to evaluate its sustainability performance and identify optimal solutions for achieving its sustainability goals. We will implement it using MATLAB as a decision analysis and decision making tool. We will further enhance the methodology through conducting more case studies. In addition, we will extend our research scope to digital twinning for sustainable metal finishing through the development of digital models for characterizing the sustainability performance of electroplating systems.
4. References
1. I. Kuntay, K. Uygun, Q. Xu and Y. Huang, "Environmentally Conscious Hoist Scheduling in Material Handling Processes," Chem. Eng. Comm., 193, 273-293 (2006).
2. J. Xiao and Y. Huang, “Technology integration for sustainable manufacturing: an applied study on integrated profitable pollution prevention in surface finishing systems,” Industrial & Engineering Chemistry Research, 51 (35), 11434-11444 (2012).
3. Q. Xu, A. Telukdarie, H.H. Lou, and Y. Huang, “Integrated electroplating system modeling and simulation for near zero discharge of chemicals and metals,” Industry & Engineering Chemical Research, 44 (7), 2156-2164 (2005).
4. Y.H. Yang, H.H. Lou, and Y. Huang, “Optimal design of a water reuse system in an electroplating plant,” Plating and Surface Finishing, 86 (4), 80-84 (1999).
5. Y. Huang, “Toward Dynamic Sustainability Assessment in the Digital Age,” Clean Technologies and Environmental Policy, 24 (9), 2655-2657 (2022); https://doi.org/10.1007/s10098-022-02416-9.
5. Past project reports
1. Quarter 1 (April-June 2020): Summary: NASF Report in Products Finishing; NASF Surface Technology White Papers, 84 (12), 14 (September 2020); Full paper: http://short.pfonline.com/NASF20Sep1
2. Quarter 2 (July-September 2020): Summary: NASF Report in Products Finishing; NASF Surface Technology White Papers, 85 (3), 13 (December 2020); Full paper: http://short.pfonline.com/NASF20Dec1
3. Quarter 3 (October-December 2020): Summary: NASF Report in Products Finishing; NASF Surface Technology White Papers, 85 (7), 9 (April 2021); Full paper: http://short.pfonline.com/NASF21Apr1.
4. Quarter 4 (January-March 2021): Summary: NASF Report in Products Finishing; NASF Surface Technology White Papers, 85 (11), 13 (August 2021); Full paper: http://short.pfonline.com/NASF21Aug1.
5. Quarter 5 (April-June 2021): Summary: NASF Report in Products Finishing; NASF Surface Technology White Papers, 86 (1), 19 (October 2021); Full paper: http://short.pfonline.com/NASF21Oct2
6. Quarter 6 (July-September 2021): Summary: NASF Report in Products Finishing; NASF Surface Technology White Papers, 86 (4), 19 (January 2022); Full paper: http://short.pfonline.com/NASF22Jan3
7. Quarter 7 (October-December 2021): Summary: NASF Report in Products Finishing; NASF Surface Technology White Papers, 86 (7), 17 (April 2022); Full paper: http://short.pfonline.com/NASF22Apr2
8. Quarter 8 (January-March 2022): Summary: NASF Report in Products Finishing; NASF Surface Technology White Papers, 86 (10), 17 (July 2022); Full paper: http://short.pfonline.com/NASF22Jul2
9. Quarter 9 (April-June 2022): Summary: NASF Report in Products Finishing; NASF Surface Technology White Papers, 87 (1), 17 (October 2022); Full paper: http://short.pfonline.com/NASF22Oct1
6. About the Principal Investigator
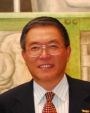
Dr. Yinlun Huang is a Professor at Wayne State University (Detroit, Michigan) in the Department of Chemical Engineering and Materials Science. He is Director of the Laboratory for Multiscale Complex Systems Science and Engineering, the Chemical Engineering and Materials Science Graduate Programs and the Sustainable Engineering Graduate Certificate Program, in the College of Engineering. He has ably mentored many students, both Graduate and Undergraduate, during his work at Wayne State.
He holds a Bachelor of Science degree (1982) from Zhejiang University (Hangzhou, Zhejiang Province, China), and M.S. (1988) and Ph.D. (1992) degrees from Kansas State University (Manhattan, Kansas). He then joined the University of Texas at Austin as a postdoctoral research fellow (1992). In 1993, he joined Wayne State University as Assistant Professor, eventually becoming Full Professor from 2002 to the present. He has authored or co-authored over 220 publications since 1988, a number of which have been the recipient of awards over the years.
His research interests include multiscale complex systems; sustainability science; integrated material, product and process design and manufacturing; computational multifunctional nano-material development and manufacturing; and multiscale information processing and computational methods.
He has served in many editorial capacities on various journals, as Co-Editor of the ASTM Journal of Smart and Sustainable Manufacturing Systems, Associate Editor of Frontiers in Chemical Engineering, Guest Editor or member of the Editorial Board, including the ACS Sustainable Chemistry and Engineering, Chinese Journal of Chemical Engineering, the Journal of Clean Technologies and Environmental Policy, the Journal of Nano Energy and Power Research. In particular, he was a member of the Editorial Board of the AESF-published Journal of Applied Surface Finishing during the years of its publication (2006-2008).
He has served the AESF and NASF in many capacities, including the AESF Board of Directors during the transition period from the AESF to the NASF. He served as Board of Directors liaison to the AESF Research Board and was a member of the AESF Research and Publications Boards, as well as the Pollution Prevention Committee. With the NASF, he served as a member of the Board of Trustees of the AESF Foundation. He has also been active in the American Chemical Society (ACS) and the American Institute of Chemical Engineers (AIChE).
He was the 2013 Recipient of the NASF William Blum Scientific Achievement Award and delivered the William Blum Memorial Lecture at SUR/FIN 2014 in Cleveland, Ohio. He was elected AIChE Fellow in 2014 and NASF Fellow in 2017. He was a Fulbright Scholar in 2008 and has been a Visiting Professor at many institutions, including the Technical University of Berlin and Tsinghua University in China. His many other awards include the AIChE Research Excellence in Sustainable Engineering Award (2010), AIChE Sustainable Engineering Education Award (2016), the Michigan Green Chemistry Governor’s Award (2009) and several awards for teaching and graduate mentoring from Wayne State University, and Wayne State University’s Charles H. Gershenson Distinguished Faculty Fellow Award.
* Dr. Yinlun Huang, Professor
Dept. of Chemical Engineering and Materials Science
Wayne State University
Detroit, MI 48202
Office: (313) 577-3771
E-mail: yhuang@wayne.edu
RELATED CONTENT
-
Nanostructure of the Anodic and Nanomaterials Sol-Gel Based Materials Application: Advances in Surface Engineering
Porous alumina can be fabricated electrochemically through anodic oxidation of aluminum. This paper reviews sol-gel chemistry and applications, which also offers unusual nanoporous microstructures. The ability to control pore chemistry at different scales and geometries, provides excellent bioactivity, enabling the entrapment of biologically active molecules and their controllable release for therapeutic and medical applications.
-
Mechanical Properties of Electroformed Metals
In 1996, the AESF held its highly regarded electroforming course, prepared by Ron Parkinson for presentation by The Nickel Development Institute (NiDI) and the AESF. What follows is a slightly modified excerpt, specifically on the mechanical properties of electroformed metals. Much of this information has withstood the test of time, and gives a perspective of this technology at the turn-of-the-century.
-
The Adhesion of Electrodeposits to Plastics
The 1966 Carl E. Huessner Gold Medal Award was given to Dr. Edward Saubestre and co-workers for Best Paper appearing in Plating in 1965, and their paper is republished here in a series on the AES/AESF/NASF Best Paper Awards. This paper is a comprehensive treatise on the Jacquet peel test, a primary test method for determining adhesion on plated plastics.